Whether a material exhibits ductility or brittleness determines its suitability for various applications. Ductility is the ability of a material to deform under stress before fracturing, while brittleness is the tendency of a material to fracture with little or no plastic deformation. In this article, we will explore the differences between ductile and brittle materials, the factors influencing their mechanical properties, and the characterization techniques used to determine their behavior under stress.
Understanding Material Deformation
Material deformation is a critical aspect of engineering that describes how materials behave under stress. Whether a material exhibits ductility or brittleness determines its suitability for various applications and can significantly influence design decisions.
Ductility
Ductility is a material’s ability to undergo significant plastic deformation before rupture. It enables materials to absorb a considerable amount of strain, facilitating their usage in applications that require bending and shaping, such as metal forming processes.
For instance, metals like copper and aluminum retain their ductility even at room temperature, allowing for intricate manufacturing processes without fracturing. Materials that exhibit ductility typically show a smooth, elongated strain response when stress is applied, indicating their ability to sustain deformation. This can be measured by percent elongation at the time of fracture:
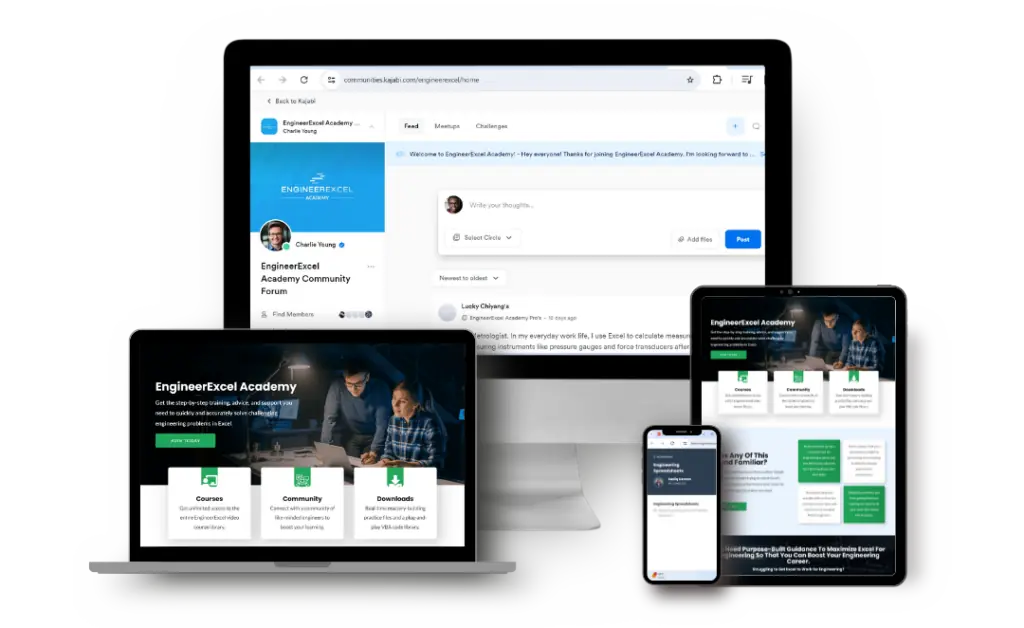
Elevate Your Engineering With Excel
Advance in Excel with engineering-focused training that equips you with the skills to streamline projects and accelerate your career.

Where:
- Lo = initial length of a specimen [m]
- Lf = final length of the specimen after deformation [m]
Alternatively, percent reduction in area within the region of necking is another way to measure ductility:

Where:
- Ao = original cross-sectional area of the specimen [m2]
- Af = cross-sectional area at the neck at fracture [m2]
Brittleness
In contrast, brittleness is characterized by a material’s tendency to fracture without undergoing any noticeable plastic deformation. Brittle failure often occurs suddenly and without warning, which can be particularly hazardous in structural applications.
For instance, cast iron, despite its high compressive strength, is prone to brittle fracture due to its relatively low tensile strength and the presence of microscale flaws. Brittle materials exhibit a steep stress-strain response until the point of failure, reflecting their inability to deform plastically when subjected to high stress levels.
Mechanical Properties
Stress-Strain Behavior
When materials are subjected to stress, their response is measured by strain. Ductile materials exhibit significant plastic deformation before failure, which is evident in their stress-strain curves as a smooth transition from the linear elastic region to non-linear plastic deformation, followed by necking and eventual fracture. In contrast, brittle materials typically display little to no plastic deformation with a sudden onset of fracture after the elastic limit is reached.
These are illustrated in the diagram below.
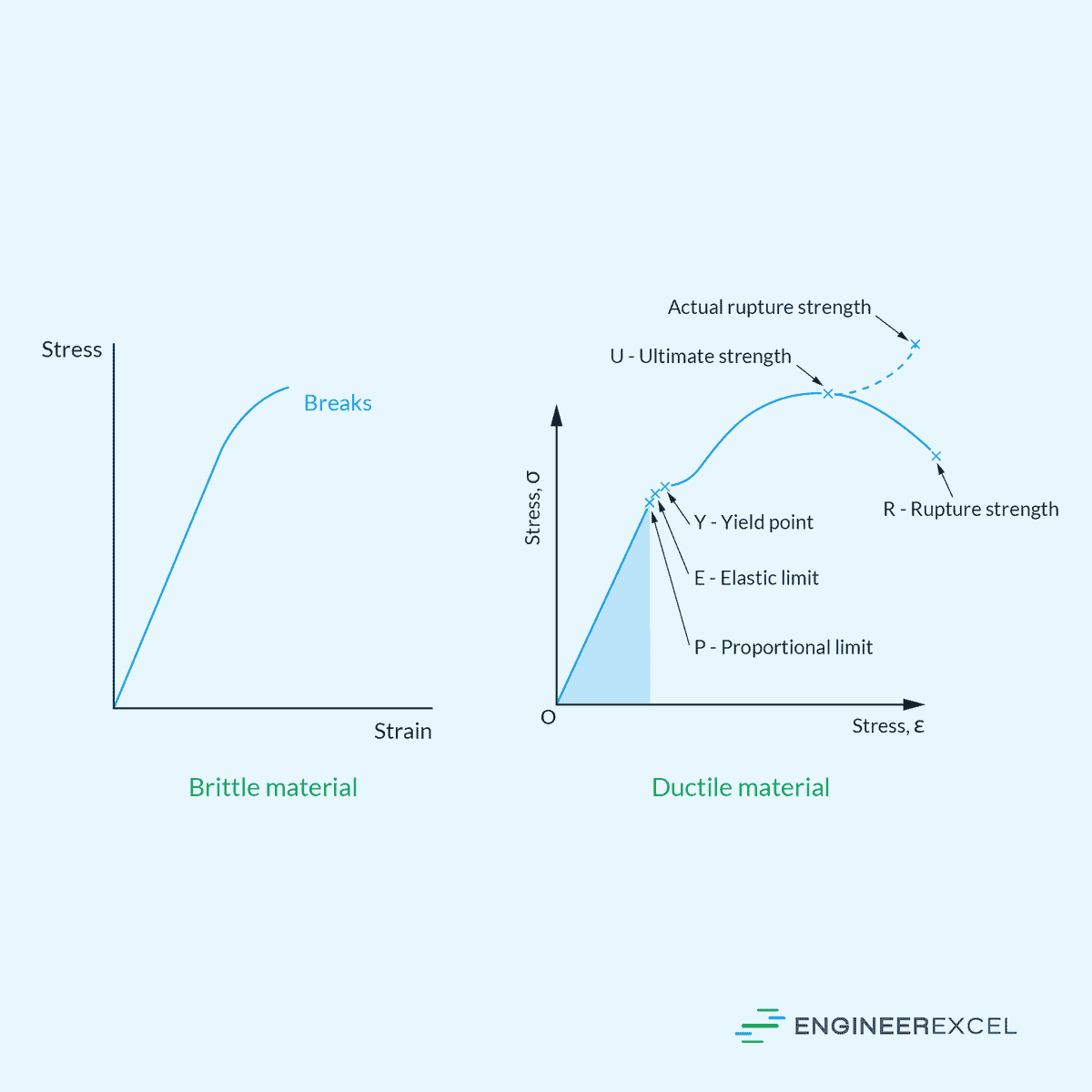
Fracture Toughness
Fracture toughness, or modulus of toughness, is the ability of a material to resist crack propagation. This is represented by the entire area under the stress–strain diagram, as shown in the diagram below.
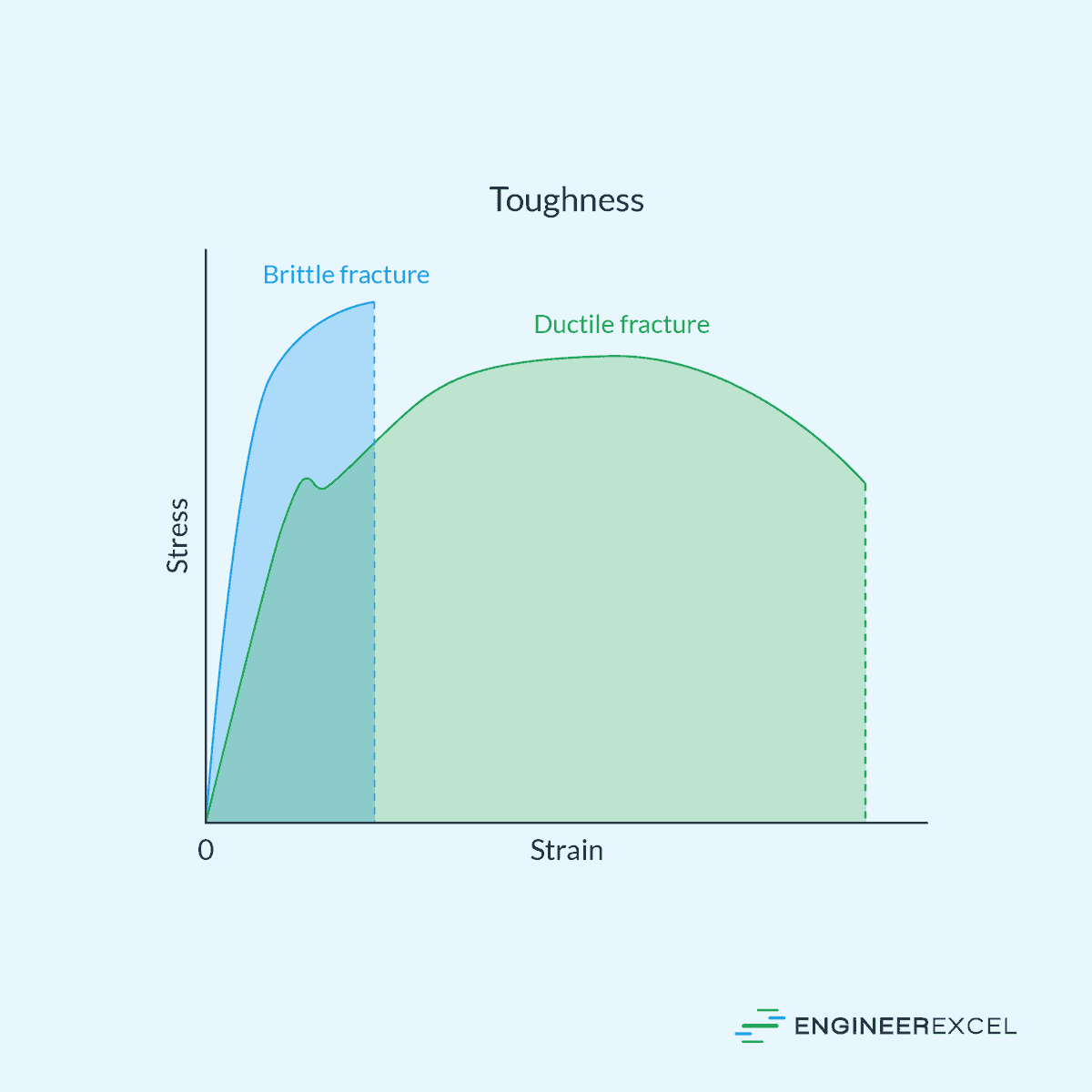
In essence, toughness represents the strain-energy density of the material just before it fractures.
Ductile materials often show higher fracture toughness, which is a critical trait for applications where resistance to fracture is vital. Brittle materials, however, can fail abruptly due to their low fracture toughness, as they’re unable to absorb significant energy before fracturing, which is demonstrated through brittle fracture mechanisms such as cleavage and intergranular cracking.
Strain Rate Sensitivity
Strain rate sensitivity is an indicator of how the strength of a material changes with the rate of loading. Ductile materials often become tougher at higher strain rates, lending them greater impact resistance. Brittle materials, on the other hand, may become more prone to fracture when subjected to rapid loading, and their lack of ductility is especially problematic at high strain rates as they’re unable to deform plastically to dissipate energy.
Factors Influencing Ductility and Brittleness
The mechanical properties of materials such as ductility and brittleness are influenced by a range of factors, from the intrinsic characteristics of the material itself to the conditions to which it is subjected.
Material Composition
Material composition directly impacts a material’s ductility or brittleness. For example, metals with a high purity often exhibit greater ductility due to fewer obstruction points within their crystal lattice. Impurities can act as points of stress concentration, increasing brittleness. The presence of certain alloying elements can either enhance or reduce ductility.
Temperature
Temperature has a significant effect on mechanical behavior. As temperatures decrease, materials tend to become brittle, as seen in materials like mild steel which retain ductility until approximately -180°C, after which ductility sharply declines. Conversely, increasing temperatures typically decrease materials’ yield strength but may increase ductility up to a point, beyond which creep deformation can lead to failure.
Grain Size
The grain size of metals can also determine the balance between ductility and brittleness. Finer grains often enhance strength and hardness due to the increased area of grain boundaries, which can impede the movement of dislocations. Large grains can enhance creep resistance at high temperatures but generally decrease toughness and increase the likelihood of distortion during processing.
Heat Treatment
Heat treatment processes modify the microstructure of materials, thus affecting their ductility and brittleness. Proper heat treatment can relieve internal stresses and refine the grain structure, thereby improving machinability, toughness, and resistance to corrosion. Different heat treatment techniques can tailor material properties to specific applications, ranging from fine-grained configurations favoring toughness to coarse-grained ones for improved workability.
Atmospheric Exposure
Finally, atmospheric exposure influences ductility and brittleness through processes such as oxidation, which can build a protective film on a metal’s surface but may also generate local cells through cracks and discontinuity, affecting electrical resistivity. Environmental conditions containing moisture or corrosive substances like sulfur dioxide can worsen these effects, decreasing overall material ductility.
Characterization Techniques
There are several characterization techniques that can be performed to determine whether a material will behave in a brittle or ductile manner under stress. Such methods provide quantitative data that informs the selection and usage of materials in various applications.
Tensile Testing
Tensile testing is a fundamental technique used to assess a material’s strength and ductility. The test measures the force required to stretch a material to its breaking point. Engineers plot this force against the material’s elongation to produce a stress-strain curve. The curve’s profile indicates whether a material will exhibit brittle or ductile characteristics under tension.
Impact Testing
Impact testing evaluates a material’s capacity to absorb energy during fracture at high strain rates. The most common forms are the Charpy and Izod tests, which involve striking a notched specimen with a pendulum hammer and measuring the energy absorbed in the fracturing process. Lower absorbed energy suggests brittleness, whereas higher energy absorption indicates ductility.
Fractographic Analysis
Fractographic analysis is used to examine the fracture surface of a material after failure. It provides insights into the material’s failure mode by revealing patterns and features on the fracture surface. Through techniques such as scanning electron microscopy (SEM), engineers can determine if the failure was due to brittle fracture, indicated by a smooth surface, or ductile fracture, often characterized by a rough surface with telltale signs of plastic deformation.
Examples of Ductile and Brittle Materials
Ductile Materials
Ductile materials, such as mild steel, are characterized by their ability to withstand significant bending and deforming without breaking. Mild steel, for example, is frequently used in structural applications due to its high toughness and capacity to undergo plastic deformation. They absorb considerable energy before failure.
Other examples of ductile materials include aluminum and copper, which are used in various applications from electrical wiring to aircraft structures due to their malleable nature.
Brittle Materials
By contrast, brittle materials such as glass and cast iron exhibit very little plastic deformation before failure. Cast iron, for instance, can withstand high compressive loads but is vulnerable to tensile stress due to the existence of microscopic flaws. These flaws lead to crack propagation when stressed, resulting in sudden fracture.
Similarly, ceramics and concrete are considered brittle as they tend to break suddenly under stress without significant prior deformation, making them suitable for applications where compressive strength is more critical than tensile strength.